Research
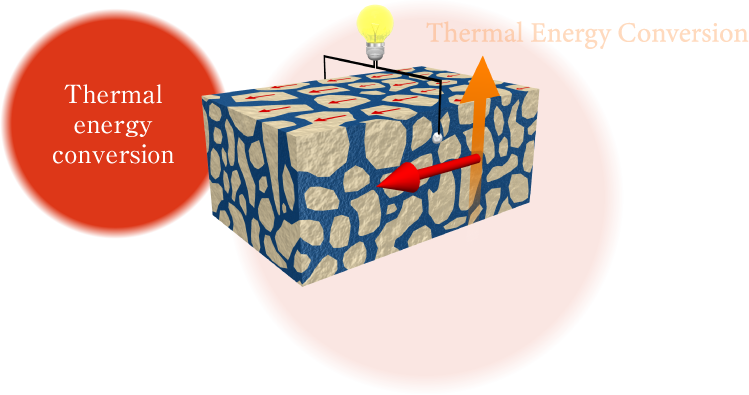
The goal of this research is to create thermoelectric permanent magnets capable of efficient transverse thermoelectric conversion with no need for applied magnetic fields. Conventional techniques require specially constructed modules in which two distinct thermoelectric materials are conjoined in complicated ways. In transverse thermoelectric conversion, by contrast, charge and heat currents flow in orthogonal directions; consequently, only one type of material is required, eliminating the headache of conjoining materials and allowing conversion modules to be highly durable and general-purpose. At present, as many as six distinct physical principles are known to furnish driving mechanisms for transverse thermoelectric conversion; unfortunately, in each case the thermoelectric conversion performance is far lower than that of conventional approaches based on the Seebeck effect.
Our research studies the design of multi-hierarchical structures capable of taking full advantage of various types of transverse thermoelectric conversion; the transverse thermoelectric performance we achieve in this way exceeds that of existing materials by at least an order of magnitude, and we are working to synthesize bulk transverse thermoelectric materials capable of operating without applied magnetic fields. In addition to synthesizing materials and studying their physical properties, we are also developing modules to exploit the advanced functionality of these transverse thermoelectric converters.
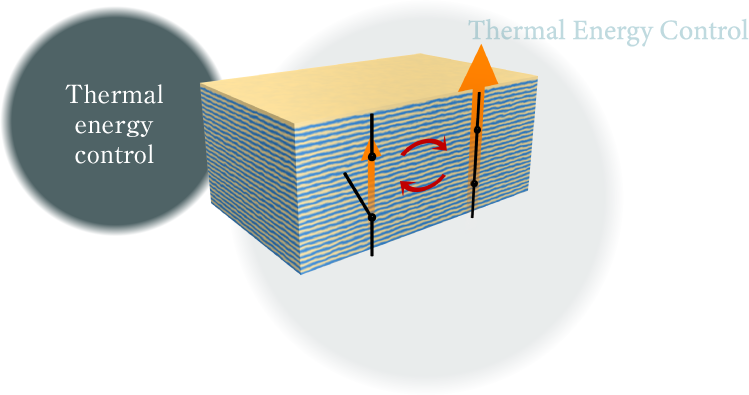
The goal of this research is to create magnetic hybrid thermal switching materials whose thermal conductivity varies dramatically depending on the direction of the intrinsic magnetization and the strength of the magnetic field. Developing the ability to modulate thermal conductivity in the presence of external fields would be a major technological breakthrough that could enable the development of advanced thermal management techniques capable of active switching between thermal conducting and insulating behaviors; for this reason, the goal of achieving thermal switching with significant change in thermal conductivity is a focus of basic research efforts in laboratories around the world.
In 2021, working with magnetic multilayer films—the basic workhorse devices of spintronics—we observed giant thermal switching (the magnetothermal resistance effect), depending on the direction of the magnetization, with the thermal conductivity changing by 25 W/mK and the change ratio of 150%. This effect works across a wide temperature range and the application of even small magnetic fields suffices to induce dramatic changes in thermal conductivity. Unfortunately, the effect also suffers from one major drawback: to date, such remarkable behavior has only been observed for thin-film samples at the nanoscale.
The goal of this research is to achieve precision control of phase interfaces and precipitate deposits in magnetic hybrid thermal switching materials—and to custom-design spin configurations and electronic conductivity distributions in layered substances—to achieve magnetically-controllable giant thermal switching effects in bulk materials at the macroscale.
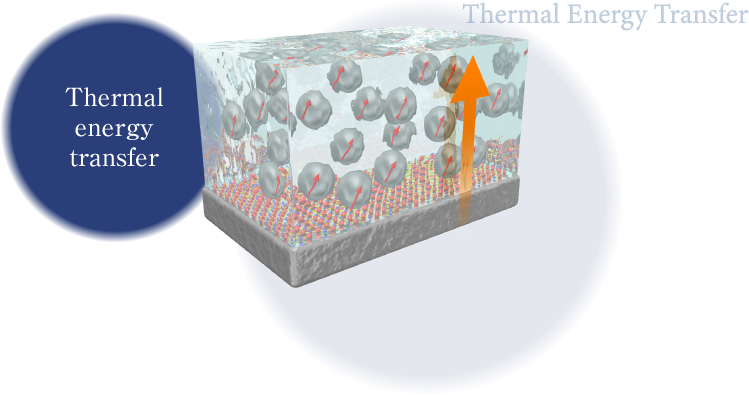
The goal of this research is to create phase-interface-controlled magnetic refrigeration materials capable of efficiently transferring heat—specifically, heat absorbed or emitted due to applied magnetic fields—to thermal media. The magnetocaloric effect—in which application or removal of a magnetic field induces heat absorption or emission—offers the potential basis for advanced temperature-control technologies requiring no coolant gas. Our focus in this project is not restricted to bulk properties of magnetocaloric materials; we also think carefully about the optimal choices of materials and coolant media for refrigeration cycles—which are of great practical importance—and we place particular emphasis on techniques for controlling the relevant interfaces, thus contributing to the development of magnetic refrigeration technologies. Our primary tools for attacking these problems are active thermal measurement techniques, the primary drivers of the dramatic recent progress in spin caloritronics—a field which, thus far, has evolved completely independently of magnetic-refrigeration research.