About us
ERATO Uchida Magnetic Thermal Management Materials Project is dedicated to creating a new class of advanced energy materials—magnetic thermal management materials—with intrinsic capabilities for using magnetism to control, convert, and transfer thermal energy at the macroscale. Although techniques and principles for thermo-spin conversion and thermal energy control using spins have been known for some time, to date they have only been effectively utilized at the nanoscale; the challenge of scaling up to practically useful system sizes has remained stubbornly out of reach. The spirit of our project is to remain undaunted by this challenge—and unshackled from the staid dogmas of conventional wisdom: by fusing multi-hierarchical structures and precision-designed phase interfaces with advanced techniques for spatiotemporal thermal measurements, we seek to demolish the barriers between the macro- and micro-worlds and develop new functional materials enabling highly efficient energy utilization at the macroscale.
Our project actively pursues joint R&D projects with corporate partners while supervising the research of graduate students and encouraging them to interact with the broader research community. If you are curious about our research—and especially if you are a student or early-career researcher interested in joining our team—please do not hesitate to contact Research Director Ken-ichi UCHIDA (Please enable JavaScript) for more information. Please also check our project website to see current job openings for postdoctoral researchers and staff scientists.
Message from
Research Director
ERATO Research Director,
Japan Science and Technology Agency
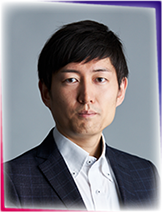
Ken-ichi UCHIDA
Distinguished Group Leader, Spin Caloritronics Group
Research Center for Magnetic and Spintronic Materials, National Institute for Materials Science
Professor, Department of Advanced Materials Science
Graduate school of Frontier Sciences, The University of Tokyo
Magnetic materials play key roles in a wide range of modern technologies—from electric-vehicle motors to power plants—and have become essential components of modern daily life. The dream that inspired this project arose from a simple question: What kind of applications can be realized if magnets—so ubiquitous in our everyday lives—could somehow be made to convert heat directly into electric power, to cool bodies by applying currents or magnetic fields to them, or to actively control heat flows? This simple question has a simple answer: Such capabilities would enable revolutionary new energy-conservation and energy-production technologies.
To turn this dream into reality, we are developing a new class of advanced energy materials that we call magnetic thermal management materials (MTMMs). More specifically, at present we are working to create—and deploy for practical applications—three types of MTMMs: thermoelectric permanent magnets for thermal-energy conversion, magnetic hybrid thermal switching materials for thermal-energy control, and phase-interface-controlled magnetic refrigeration materials for thermal-energy transfer. The key insights needed to create functional MTMMs come from the field of spin caloritronics, which combines spintronics—a strong candidate for the next generation electronics—with the thermoelectric and thermal-transport properties of materials. By infusing spin caloritronics—which, to date, has existed only at the level of basic research—with techniques and insights from other disciplines, we will establish novel energy harvesting and thermal management techniques based on new physical principles in which spin—the origin of magnetism—is the driving force. If we can develop this new technology to a stage at which it can help to create sustainable future societies, our highest ambitions for this project will have been achieved.
The core mission of this project is to connect microscopic thermo-spin conversion principles—which, to date, have only been available at the nanoscale—to the macroscopic material properties. By focusing on the interplay between heat currents, charge currents, and spin currents (flows of magnetism), researchers in spin caloritronics have discovered an impressive—and growing—list of novel physical phenomena; unfortunately, most of these effects are governed by a characteristic length scale, known as the spin diffusion length, whose order of magnitude typically ranges from nanometers to micrometers. Exploiting these phenomena to control thermal energy at the macroscale will require scaling them up to the domain of bulk solids, but this has not been possible to achieve simply by extending existing methods. To transcend the barriers separating the macroscopic and microscopic worlds, we are combining methods for controlling phase interfaces with multiscale materials-science techniques based on cutting-edge insights into spin caloritronics; consequently, this field—which has heretofore been restricted to proof-of-concept experiments and exploration of individual functionalities—is now in the process of being revolutionized by the results of our research.
It is no exaggeration to say that every step in my scientific career has been taken in partnership with JST. From 2012 to 2017, I worked on the JST Strategic Basic Research Program PRESTO "Phase Interfaces for Highly Efficient Energy Utilization", conducting basic research to extend the basic principles of and increase the efficiency of the spin Seebeck effect, in which heat currents give rise to spin currents. Then, from 2017 to 2023, I worked on the JST Strategic Basic Research Program CREST "Creation of Innovative Core Technologies for Nano-enabled Thermal Management," establishing methods for measuring various spin-caloritronic phenomena in which heat is produced as an output and demonstrating their functionalities. As these accomplishments testify, spin caloritronics remains an internationally active field; however, we cannot expect progress to continue indefinitely as long as the field remains confined to the domain of basic research. We firmly believe that it is our responsibility to elevate the field to a standard allowing serious discussion of practical applications—and to lay sound groundwork for its future evolution—and we are hopeful that this project will furnish an ideal framework for these goals.
Our research team comprises six research groups. A crucial feature of our project is that our six group leaders include not only condensed-matter physicists specializing in spin caloritronics, but also researchers from a wide variety of other backgrounds, including metallurgy (permanent magnet development), nanostructure analysis, thermal measurement, and heat-transfer engineering. We are confident that this combination of multidisciplinary talent will allow us to shatter the barriers restricting conventional spin caloritronics, build new physics and materials-science platforms, and deliver the world’s first successful MTMMs.
We are grateful for your guidance and support as we explore these fascinating scientific and technological frontiers.