Overview of the Project
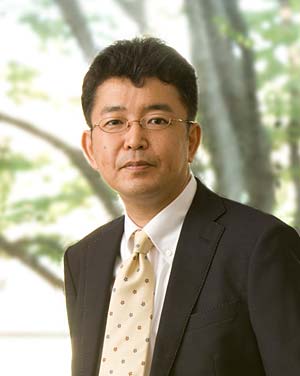
Human history has been characterized by tireless explorations of time. Almanacs compiled in Egypt by observation of celestial motion made possible the forecasting of when the next flooding of the Nile might come, enabling uninterrupted farming in the fertile land. The land then became the basis of ancient Egyptian prosperity. Following Galileo Galilei, who had discovered the isochronism of the pendulum in the 16th century, Christiaan Huygens built sophisticated pendulum clocks that became important technology in the Age of Discovery. The cesium atomic clock, an invention by Louis Essen, defined one second in 1967 and achieved one second cumulative error over several tens of millions of years. This invention was half a century ago. Clocks, through so much sophisticated evolution, have obtained higher accuracy that is seemingly unconnected with our daily life. They are, however, part of the infrastructure of today’s global society being applied to high-speed and broadband communication technology as well as highly accurate positioning technology by GPS satellites as exemplified by car navigation systems. Thus, innovation in time measurement has been a major driving force in the progress of civilization.
Since ancient times the concept of time has attracted philosophers and scientists. Isaac Newton, in the 17th century, advocated his theory of classical mechanics that describes the motion of objects we experience every day. After Albert Einstein’s publication of the theory of relativity in the 20th century, the concepts of absolute time and absolute space advocated by Newton collapsed. We saw instead the birth of relativistic space-time. Thus, changes in the concept of time have significantly influenced human cognition of nature. We recognize time by counting the number of repetitions of periodic phenomena in celestial movements or pendulum clocks. Study in time means the pursuit of more precise and stable periodic phenomena. The establishment of quantum mechanics in the 20th century was led by atomic spectroscopy that enabled us to measure frequencies of light or electromagnetic waves radiating from atoms for exploring the internal structure of the atom. Atomic spectroscopy offered an experimental basis for quantum mechanics by discovering the laws that govern microscopic particles. These achievements led to the latter-day version of the study of time - study of the atomic clock.
For more than half a century, physicists have tried to create an ideal pendulum clock using an atom that is still in free space without an electromagnetic field. The single ion optical clock proposed by Hans Georg Dehmelt, who shared the Nobel Prize in Physics in 1989, was regarded as the ultimate atomic clock, which paved the way for global research and development works However, as we were entering the present century, a limitation in time measurement due to quantum fluctuations of a single atom was identified as a practical dilemma. For instance, in order to read out an atomic clock with 18-digit precision time/frequency, which means a clock with a one second uncertainty over 30 billion years, it is necessary to continue measuring for approximately 10 days or 1 million seconds to average the effect of quantum fluctuations. Even if there is a slight time fluctuation during this averaging time, it is impossible to observe the phenomenon as it would have been averaged already. There are time domains that we are still unable to access. What if we could get millions of atoms that are still in space and do not interact each other? Instead of the averaging the measurement for 1 million seconds, it would be possible for us to achieve 18-digit precision with a measurement for a second.
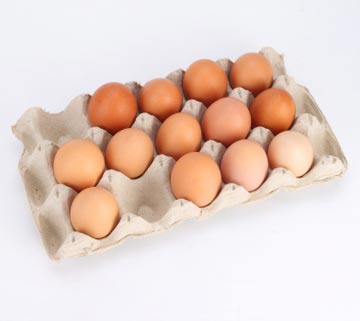
We aimed to develop a new atomic clock using an approach that defied the common sense of conventional atomic clock development. We used an electromagnetic field, which affects the atomic pendulum and therefore had been excluded in constructing accurate clocks, to create an ideal atomic pendulum by freezing the motion of atoms. This was accomplished by an optical lattice created by interference fringes of a laser. This lattice is something like an egg carton that packs atoms. An important trick here is to make this egg carton invisible to the atomic pendulum. When an optical lattice is constructed by specific laser wavelength, which we termed the Magic Wavelength, the atomic pendulum, without being affected by the existence of this egg carton, can tick with the eigen frequency of atoms. It was 2001 when we proposed the idea of this optical lattice clock.
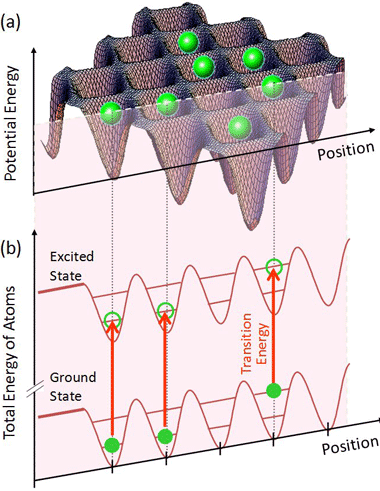
This optical lattice clock enables us to explore space-time physics. With the state-of-the-art laser cooling and trapping, quantum state control, and laser control technologies, the project will develop optical lattice clocks that will overwhelmingly exceed cesium atomic clocks in precision. By observing millions of atomic pendulums trapped in an optical lattice that is tuned to the Magic Wavelength, it would be theoretically possible to measure time of 18 digit accuracy by one-second observation. This level of uncertainty is equivalent to less than one second uncertainty after the entire cosmic age or 13.8 billion years, after the Big Bang.
This optical lattice clock will show, in real time, curvature of relativistic space time that we have never recognized in our daily life. With the precision of an optical lattice clock, it will be possible to observe how the time passage is affected by gravity just for a 1 cm height variation on the earth. When relativistic effects come into our personal scales and when they become visible in a few seconds, we would be forced to recognize how difficult for us to share the same time. A world view represented by the “distorted clocks” in Salvador Dali’s painting, La persistencia de la memoria, purportedly inspired by Einstein's theory of relativity, may become reality, and our classic recognition of space and time will be forced to change. Atomic clocks will then no longer be a means to share time with others but a probe to explore curved space time and will create a new kind of measurement technology. For instance, detecting gravitational red shift by clocks will enable us to find undiscovered natural resources or crustal movement at a subterranean level. Thus future atomic clock will become a new tool to open up new fields such as relativistic geodesy.
Foundations of both physics and the atomic clock have been based on an assumption that a physical constant is an invariable quantity. A dimensionless constant called the fine structure constant is a basic constant that scales the frequency of the atomic pendulum. Because the constant remains constant, it can be assured to tell the same time, independent of atomic elements employed in the clocks. Precise comparison of highly accurate atomic clocks consisted of different atomic elements may give us experimental means to test the constancy of the physical constants. Alternatively, if such clocks showed different time passage on the earth, where gravitational potential of the sun changes throughout the year due to its elliptical orbit around the sun, it would suggest a coupling between fine structure constant and the sun’s gravity. This kind of experimental challenge may provide an experimental basis toward a unified theory.
While we are achieving such overwhelmingly accurate time piece, it is about time for us to conceive novel applications for this new clock. When Louis Essen invented the atomic clock about half a century ago, did anyone anticipate the application of the atomic clock to car navigation systems? In front of a clock with time-resolution by 18-digit, our imagination is being questioned if it is more creative than Dali's. This project aims at the creation of new trends in quantum metrology by exploration of fundamental issues involved in the physics and engineering of relativistic space-time.
ERATO KATORI Innovative Space-Time Project started in 2010 as one of the ERATO projects (Strategic Basic Research Programs) by the Japan Science and Technology Agency. This project is carried out in cooperation with the University of Tokyo, RIKEN and the Japan Science and Technology Agency.