Progress Report
Large-scale Silicon Quantum Computer4. Quantum computing in small qubit systems
Progress until FY2022
1. Outline of the project
The project aims to demonstrate quantum algorithms using a small number of high-fidelity spin qubits in silicon. We unveil technical challenges in quantum operations and control techniques, which are expected to be crucial in future large-scale silicon quantum computers.
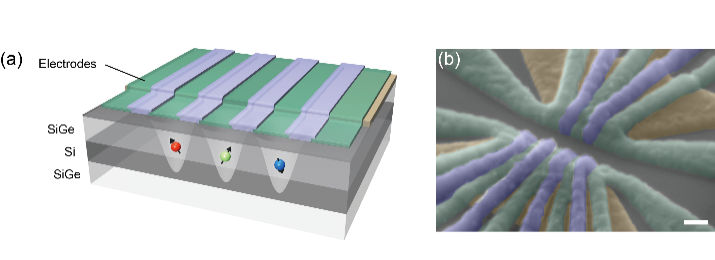
We implement quantum algorithms in Si/SiGe quantum dot devices where high-fidelity control of spin qubits has been established (Fig.1). We explore the feasibility of fault-tolerant quantum computation that requires high-fidelity initialization, readout, and coherent control.
2. Outcome so far
- ①Measurement and initialization of qubits in the middle of quantum dot arrays.
- ②Universal quantum control of three spin qubits
- ③High-fidelity two-qubit gate for fault tolerance
- ④Mitigation of control errors in high-speed manipulations
- ⑤Phase-flip error correction with three spin qubits
In ①, we have implemented initialization and readout of a spin qubit in the middle of a quantum dot array using SWAP operations. This technique allows us to scale up the system to a longer array where many qubits are not directly accessible from reservoirs.
We have then demonstrated universal control of three spin qubits in ②. We have generated a three-spin entangled state (GHZ state) with a fidelity of 88%.
We have also investigated high-fidelity two-qubit control in③ and ④. By optimizing the speed of quantum gates and the effect of noise, we have realized a controlled-NOT gate with a fidelity as high as 99.5% which is believed to be sufficient for fault-tolerant quantum computation (Fig.2).
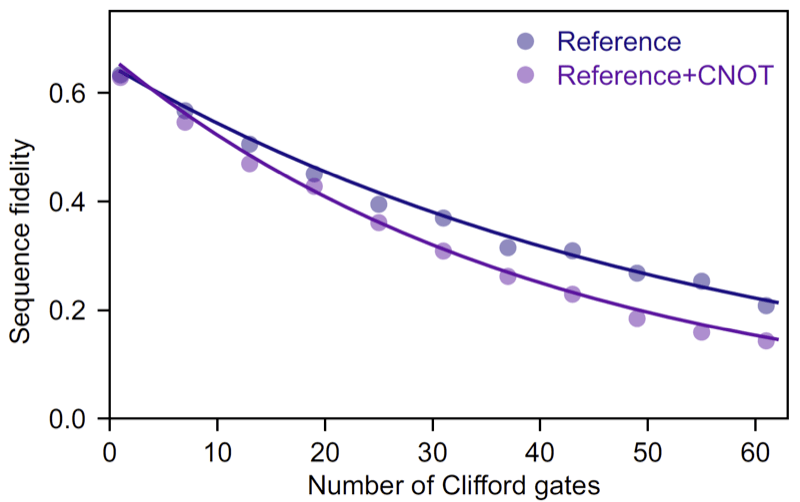
Finally, we have demonstrated quantum error correction using a three-qubit phase-flip code in ⑤. This is a big step toward fault-tolerance silicon quantum computers.
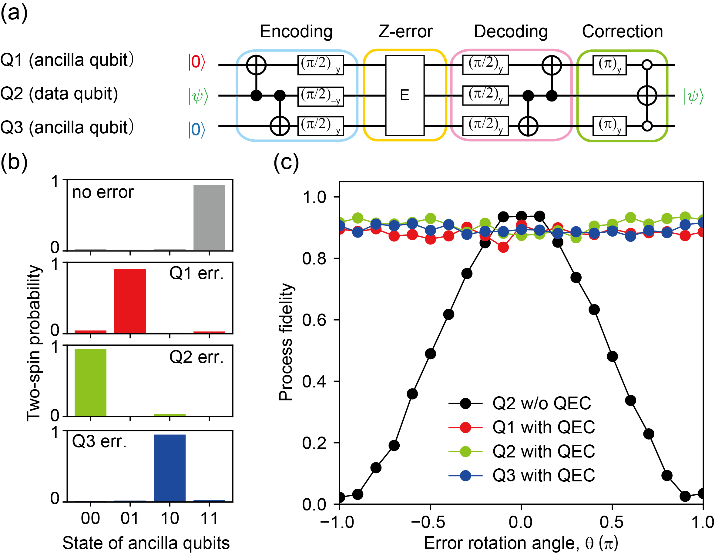
3. Future plans
Practical quantum error correction requires fast, high-fidelity qubit readout in addition to high-fidelity control. Since the readout of silicon spin qubits is orders of magnitude slower than control, we plan to improve it using the RF reflectometry technique and the Pauli spin blockade phenomenon. We further explore the feasibility of larger arrays of silicon spin qubits by connecting distant qubits via electron shuttling.