Progress Report
Fault-tolerant Quantum Computing with Photonically Interconnected Ion Traps4-2. R&D for Cloud Quantum Computing
Progress until FY2022
1. Outline of the project
We will implement automation and remote operation technologies that are essential for the cloud computing of ion trap quantum computers and create an environment where small-scale quantum computers can be used remotely by educational institutions and other organizations. To this end, we will greatly improve the reliability of basic ion control technology and enable all series of operations in experiments (e.g., ion loading, laser cooling, gate operation, etc.) to be performed remotely. Furthermore, we will realize a quantum computer with a relatively small number of ions (on the order of 10) using established ion internal state qubits and provide an environment in which these can be operated remotely. In addition, as fundamental technology development to improve the performance of the cloud-based ion trap quantum computer, we will conduct ion transport experiments to integrate the ion trap module and two-dimensional ion array experiments to expand the number of qubits.
2. Outcome so far
For the cloud-based ion trap quantum computer, we set up the ion trap system. For the ion trap system used for laser cooling, a machined 3D linear trap, an atomic oven, and other equipment were installed in the vacuum chamber (Fig. 1), and the entire vacuum system was baked and the optics and optical components for cooling were placed on the optical table (Fig. 2). Frequency stabilization of the semiconductor laser source (935 nm) for cooling was also performed. Using an optical frequency comb generator as a reference for frequency stabilization and evaluating the frequency with an optical wavelength meter, we found that the fluctuations were within 1.6 MHz during 40 h of operation. This indicates that the continuous operation time and stability are sufficient to withstand operation in cloud computing for more than 24 hours. In addition, we have designed and installed optics for stimulated Raman excitation, stabilized the repetition rate of a picosecond pulsed laser, which is necessary for stable qubit control using the stimulated Raman transitions, and succeeded in phase-locking a beat signal around 1 GHz. In the future, we will modify the pulsed laser to perform phase locking on beat signals corresponding to hyperfine structure spacing (around 13 GHz).
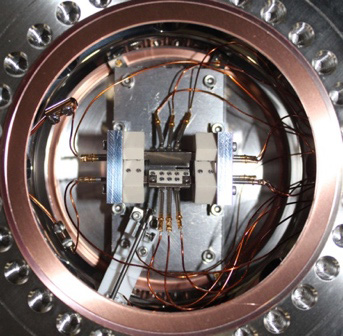
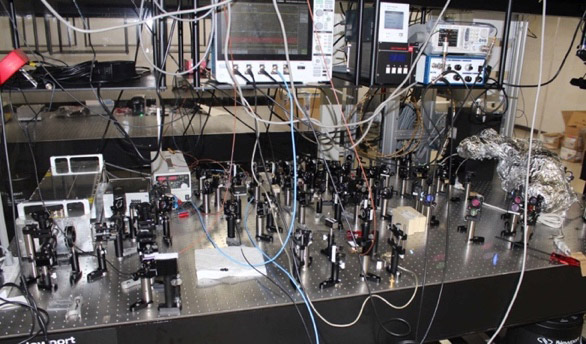
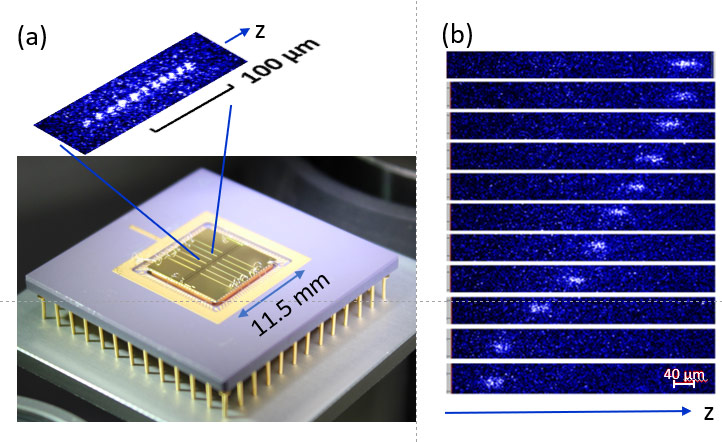
We perform experiments of ion transportation using a surface-electrode trap which consists of multiple trapping regions as shown in Fig. 3 (a). We manipulate the trapping potential by varying voltages applied to these electrodes. We have derived appropriate voltages by use of a method for solving optimization problems so that we move the ion position with maintaining the potential curve around the ions. Figure 3 (b) shows one of the experimental results. Around the starting (ending) position, we accelerate (decelerate) the ions slowly to avoid heating ions.
3. Future plans
We will implement a quantum gate, improve its accuracy, and implement a relatively simple algorithm so that the quantum gate algorithm experiment can be operated. Ion transportation technique is of significance because it determines the computation time of quantum computers. We will develop the faster transportation technique based on the above-mentioned results.