Research Results
Verification of the theory of relativity to the estimation of underground resources
Optical lattice clock changes our concept of time FY2016
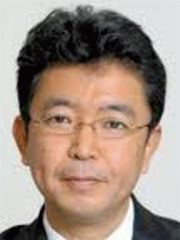
- Hidetoshi Katori (Professor, University of Tokyo/Senior Research Engineer, RIKEN)
- ERATO
- "KATORI Innovative Space-Time Project" Research Director (2010-2016)
Precision of an optical lattice clock is 1,000 times higher than the current International Atomic Time
There is a clock called the "optical lattice clock." First, each atom cooled by laser is captured in a 3-D micro space (optical lattice), which is created by interference of laser beams with special wavelength called the "magic wavelength," to prevent interaction between the atoms. Next, these atoms are irradiated with another laser beams, and the frequency of the absorbed light (resonance frequency) is measured precisely to determine the length of a second. Since an optical lattice as a whole can capture approximately one million atoms, the time can be determined in a short time by measuring their resonance frequencies all at once, and then taking the average.
This optical lattice clock is a major candidate for the "definition of a second" of the next generation. The accuracy of the cesium atomic clock, which is the current universal time, has an uncertainty of 10-15, or 1 second per 30 million years. Researchers across the world aim to improve this by approximately 1,000 times to achieve higher precision using the method mentioned above.
The research group led by Professor Hidetoshi Katori, an inventor of the optical lattice clock, developed the two optical lattice clocks, which perform the high- precision spectroscopy of strontium atoms under a low temperature environment through the research process of ERATO and proved the statistical agreement at a fractional uncertainty of 2 × 10-18 between those two clocks in May 2014. This research group newly developed the optical lattice clock based on mercury atoms and directly compared it with a low temperature operation strontium-based optical lattice clock, which has the world's best accuracy, in March 2015. The frequency ratio of mercury and strontium was obtained in a high accuracy significantly better than the second currently defined as unit of time. This achievement may accelerate the movement toward the future redefinition of a second. In June 2014, the research group also has succeeded in high-precision spectroscopy of strontium atoms in a hollow optical fiber. This is a significant achievement that will be a new foundation technology toward downsizing the quantum measurement instrument. Their goal for the future is to develop the optical lattice clock in a small size.
Pattern diagram of optical lattice
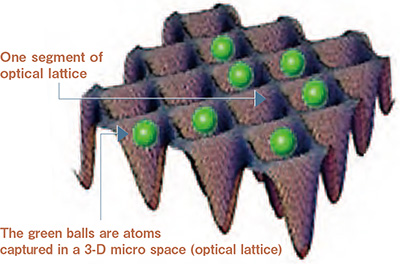
Create an optical lattice in a hollow fiber to confine strontium atoms
The research group, which has been advancing technological development of downsizing the optical lattice clock, focused their attention on a hollow core photonic crystal fiber (hereinafter referred to as "hollow core fiber"). Since a hollow optical fiber can confine light and atoms in a hollow core, it was considered to be a prospective downsizing technology.
However, in the previous expectation, because of interaction among atoms and between atoms and the hollow core fiber wall, resonance frequencies expanded to more than several MHz. Therefore, it was considered that obtaining narrow spectra close to natural linewidth was difficult in optical fibers.
The research group created an optical lattice with magic wavelength in the hollow core fiber, and captured each laser-cooled strontium atom into each segment of the lattice, in order not to broaden the linewidth of the atom's resonance spectra.
To create a high-precision optical lattice clock, there needs to be sufficient time for interrogating the clock transition. Thus, they attempted to prevent collision between strontium atoms and residual gas in the fiber. As a result of measuring the lifetime for capturing atoms in the fiber, it was confirmed that the trap lifetime was 350 to 500 milliseconds, which was sufficient to construct an optical lattice clock.
Overview of experiment apparatus
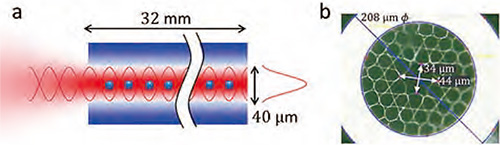
In a hollow fiber with a core diameter of 40 μm and length of 32 mm, create an optical lattice with magic wavelength (red wave line) and capture strontium atoms (blue ball). Constitute a 1-D optical lattice in the hollow core fiber. The distance between strontium atoms becomes half of the magic wavelength (approximately 0.4 μm).
A sectional view of the hollow fiber that was used. The central part is hollow.
Reduce spectral linewidth to near 1/1,000
To reduce quantum noise of an atomic clock, it is important to increase the number of atoms that are observed. Since laser beam expands by diffraction in free space, atoms can be captured only in an area near its focal point. To the contrary, in a hollow core fiber, light can be transmitted without expanding its beam size inside the core. Therefore, it is possible to greatly increase the number of atoms that are captured in proportion to fiber length.
In this case, what should be considered is whether just one atom can be captured in one segment of an optical lattice to prevent interaction among atoms. Therefore, after capturing atoms into an optical lattice and introducing them into a hollow core fiber, and the atoms are released from the optical lattice to freely expand in the hollow core fiber, then each atom is captured again. By doing so, the number of atoms in each segment of the optical lattice was reduced to one or less. With this method, the number of atoms could be maintained, while interaction among atoms was reduced. As a result, the resonance spectrum of frequency linewidth of 7.8 kHz was obtained.
Previously, spectra of atoms and molecules measured in an optical fiber was approximately 5 MHz. However, by introducing the optical lattice with magic wavelength, the spectral linewidth was reduced to 1/1,000 than that of before. The obtained spectral linewidth is close to the atomic natural linewidth, and no interaction with the fiber wall is observed. However, it was estimated by calculation that the influence of the interaction between atoms and the fiber wall on the precision of a clock is 10- 17 (uncertainty of 1 second per 3 billion years). These provide a foothold for constructing a compact and high-precision optical lattice clock using the hollow core fiber.
Expand atoms in 1-D lattice
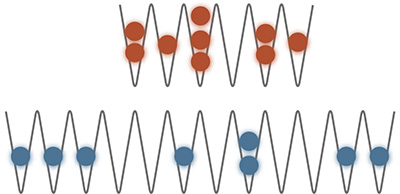
The spread of atoms in transverse direction is small immediately after introducing atoms in the fiber, and multiple atoms are captured in a segment of the lattice (the upper diagram). Interaction among atoms broaden the atomic spectrum. By capturing atoms again in the optical lattice after releasing the atoms once from the optical lattice to diffuse them, the number of atoms in each segment of the optical lattice was reduced to one or less.
Uncertainty is only 0.4 seconds per 13.8 billion years, which is the age of the universe
In an ultra precise clock, the influence of Einstein's theory of relativity, "The stronger the gravitational potential, the slower time passes," can be measured. The goal of the research group is to attain the precision of 1 × 10-18 (uncertainty of only 0.4 seconds over 13.8 billion years from the Big Bang to today, which is the age of the universe). They can detect the differences in the passage of time (according to the general theory of relativity) that is brought by gravity with an elevation difference of only 1 cm. In addition, time delay (according to the special theory of relativity) occurring at a person's walking speed can also be detected.
The gravitational field is powerful when there is mineral vein underneath and time will move more slowly than that of its preferential area. The achievement of a compact optical lattice clock enables the search for mineral resources underground. It is expected that development of technology aiming for the downsizing and improved portability of the optical lattice clock will continue based on this achievement.