Progress Report
Quantum Cyberspace with Networked Quantum Computer3. Semiconductor networking technology
Progress until FY2022
1. Outline of the project
We will develop the technologies of quantum interface between photons and silicon qubits based on spin of electrons or holes in semiconductor quantum dots (QD) and intermediate distance coupling between silicon qubits for constructing networks of semiconductor quantum computers. We also develop the connection of semiconductor qubits to large-scale optical networks. By accomplishing these research subjects, we will establish the semiconductor networking technologies for fault-tolerant quantum computers, which is the goal of Moonshot project.
Toward this goal, the detailed research subjects that we focus are the intermediate distance coupling between silicon qubits networking silicon NISQ quantum computers, Ge hole QDs as a quantum interface for telecom photons and a creation of an entanglement between remote spin qubits from an entangled photon pair.
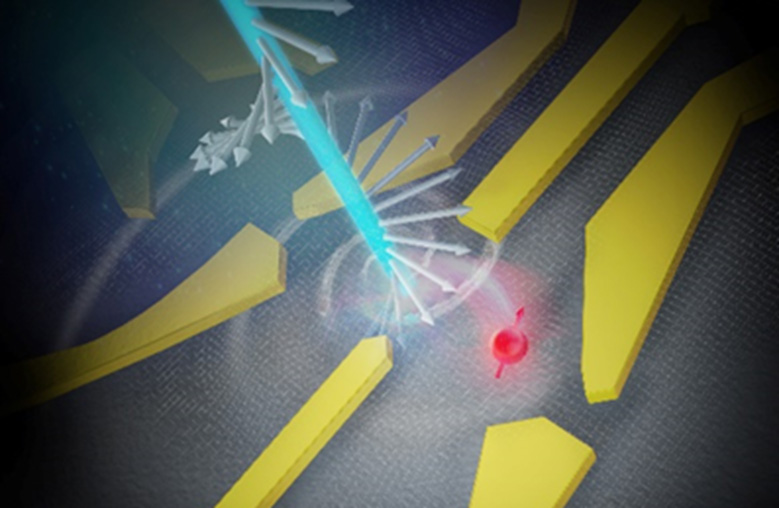
2. Outcomes so far
① Adiabatic shortcut for a semiconductor spin qubit
As an intermediate distance coupling technology, adiabatic quantum state transfer (AQT) transfers a quantum state from one end to the other by adiabatically controlling the exchange interactions at both ends in a spin chain formed in a one-dimensional QD array. However, a longer operation time is needed for a longer spin chain to prevent the diabatic error process. The adiabatic shortcut is generally known as a speed-up method. We demonstrated the accelerated adiabatic passage for single electron spin in a semiconductor qubit, for the first time, by adding a counter diabatic term. This would offer a way to speed up the AQT in the future.
② Ultrafast and high-fidelity spin manipulation using interdot tunneling in a double QD
While the single spin manipulation is conventionally performed in a single QD, we can speed up the spin manipulation by utilizing interdot tunneling in a double QD. Moreover, the Rabi decay time can be also maximized at the fastest spin manipulation condition and the fidelity for single spin control exceeds 99% which is the highest value reported in GaAs spin qubits. This result would be useful for a strong coupling of spin qubits with a superconducting resonator for the intermediate coupling between silicon qubits and photon-silicon qubit quantum interface.
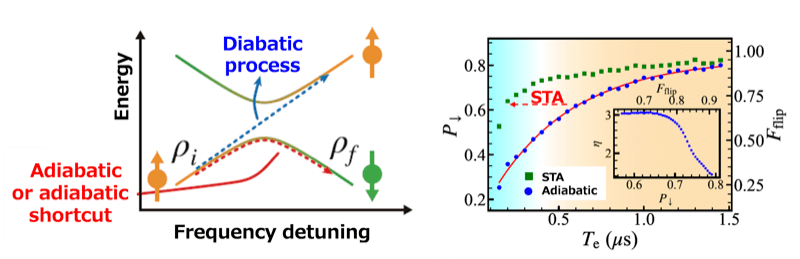
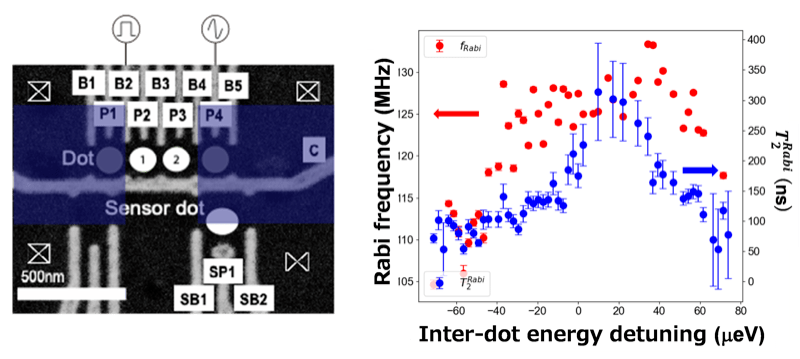
3. Future plans
Utilizing the results obtained, we will develop the intermediate coupling between Si qubits and photon quantum interface. Moreover, we will establish the photon-spin quantum interface based on Ge QD operating at telecom wavelength and introduce entangled photon pairs for networking.