Progress Report
Development of Quantum Interfaces for Building Quantum Computer Networks3. Piezoelectric Microwave Cavity
Progress until FY2022
1. Outline of the project
This project aims to develop a quantum interface that connects superconducting qubits with photons for communication to realize a large-scale distributed superconducting quantum computer. In this R&D theme, we will develop a piezoelectric microwave resonator that will be a component of the system (Fig. 1). In this R&D theme, we have achieved the following outcome.
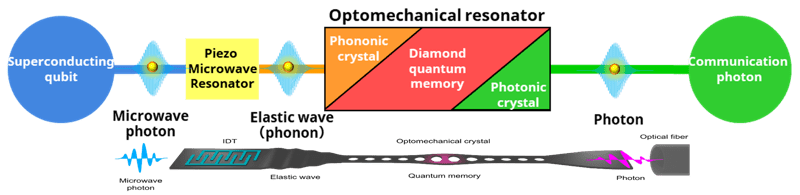
2. Outcome so far
Subject 1: Piezoelectric Microwave Cavity
- Material search and structural design of piezoelectric microwave cavity
In order to convert microwave photons into phonons with a wavelength of ~1 mm, which is equivalent to telecommunication photons with a wavelength 5 orders of magnitude shorter, we fabricated a diamond surface acoustic wave (SAW) device by forming a piezoelectric material, aluminum nitride (AlN), on diamond (Fig. 2). - Conversion from microwaves to phonons
We have succeeded in quantum manipulation of NV-centered quantum memory by generating phonons from 5 GHz microwaves (Fig. 2a). - Quantum manipulation of NV-centric quantum memory by microwaves
We have succeeded in quantum manipulation of NV-centric quantum memory by the electric field of microwaves (Fig. 2b). We have also fabricated a microwave resonator with a Q-value of 8,000.
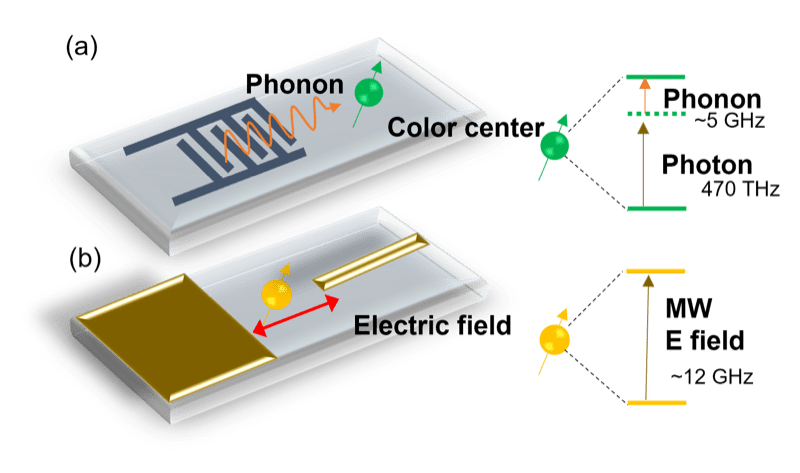
Subject 2: Quantum-Controlled Electronic Integrated Circuits
- To achieve fast and high-fidelity quantum control of quantum circuits at cryogenic temperatures, we have developed a superconducting microwave pulse generator capable of generating microwaves of arbitrary amplitude (Fig. 3). A single flux quantum (SFQ) circuit is used to generate a 100 GHz density-modulated SFQ pulse train, and a superconducting filter is used to generate a 5 GHz microwave pulse.
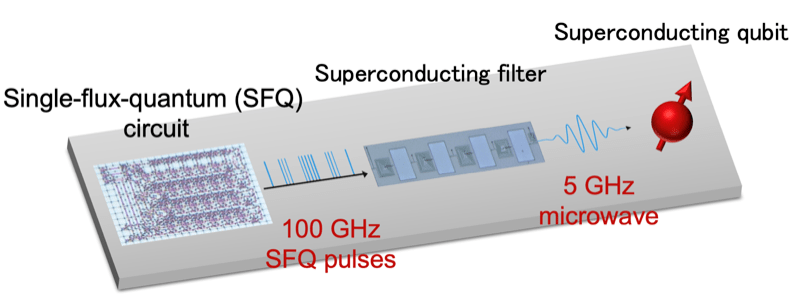
Subject 3: Theoretical study of quantum interface
- We theoretically investigated the quantum media conversion from a superconducting-atom qubit to a microwave-photon qubit. We proposed a method to demonstrate the SWAP gate between an atom qubit and a frequency-encoded photon qubit using a weak classical microwave pulse at the single photon level, and confirmed the bidirectional quantum state transfer. The fidelity of the atom-to-photon (photon-to-atom) state transfer reached 0.829 (0.801) (Fig. 4).
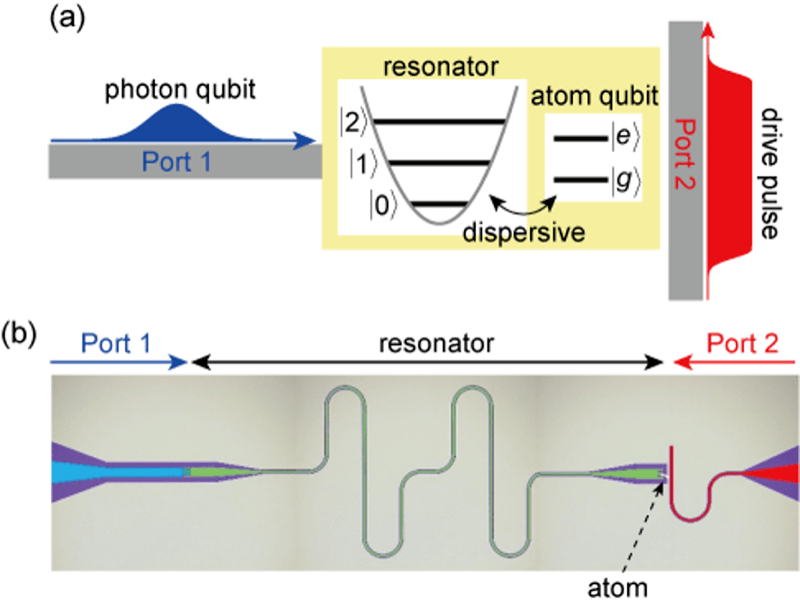
3. Future plans
We will improve the performance of piezoelectric microwave cavities and integrate them with optomechanical cavities with quantum memory.