Research Results
Dramatically extending the range of electric vehicles
Development of a revolutionary electrode material for use in lithium-air batteriesFY2017
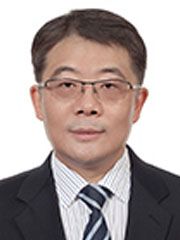
- Chen Mingwei (Professor , Tohoku University Advanced Institute for Materials Research (AIMR))
- CREST
- Specializing in phase interface science for highly efficient energy utilization
Interface science-inspired nanoporous composites for next-generation energy devices (2016-2019)
Not lithium-ion, but lithium-air batteries
Enhancing the performance of electric vehicles is seen as a promising way to reduce CO2 emissions. The range of electric vehicles is currently only about 200km, making long-distance driving difficult, and this is hindering their popularization. The issue is: How can we substantially increase battery capacitance (the amount of electricity batteries can store)?
Interest in lithium-air battery technology has increased in recent years. It is thought that a capacity of five to eight times higher than lithium-ion batteries may be achievable with lithi- um-air batteries, and worldwide competition is intensifying amongst those working on their development.
"CREST", one of the JST Strategic Basic Research Programs, is a team-based research project that advances task-oriented basic research aimed at achieving strategic targets set by the Japanese government and creating revolutionary technologies that can lead to innovations in the field of science and technology. Profes- sor Chen Mingwei and his colleagues are working on the research and development of lithium-air batteries as part of the " interface science-inspired nanoporous composites for next-gen- eration energy devices" research project in the field of "phase interface science for highly efficient energy utilization".
As part of his work in the research program, Professor Chen has succeeded in developing base materials that may lead to a revolutionary new kind of lithium-air battery.
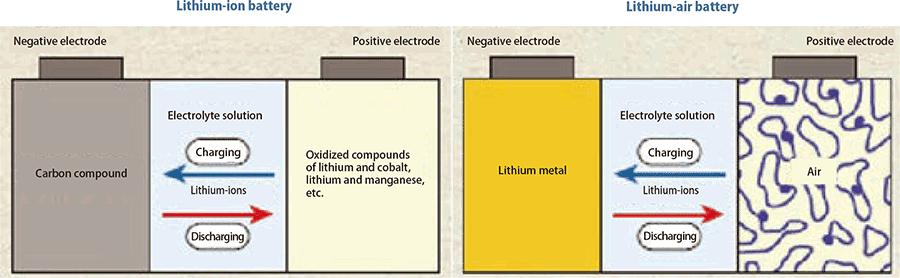
Focusing attention on nanoporous materials
Lithium-air batteries are charged and discharged through interactions between the three phase interfaces of solids, liqu ids, and gases, using lithium metal as the negative electrode and air as the positive electrode. Discharging involves causing lithium ions produced at the negative electrode to react with oxygen at the positive electrode to generate lithium peroxide, while charging involves breaking down the lithium peroxide at the positive electrode into lithium-ions and oxygen before returning them to the negative electrode. A paper in 2012 has been gar- nering a great deal of attention on the use of nanoporous gold as electrodes. Since gold is expensive, heavy, and only available in limited quantities, he decided that he would need to come up with a different material.
Folding graphene into a three-dimensional material
Amidst increasing domestic and international competition, Professor Chen and his colleagues took on the new challenge of making graphene three-dimensional—that is—creating nanoporous graphene. Graphene consists of paper-like sheets of interconnected carbon atoms. Folding this two-dimensional material into a three-dimensional material allows the creation of a material with a large surface area that takes up little space. It is the same as crumpling paper into a ball: The surface area does not change but the size is reduced. Professor Chen' s devised a solution that, instead of layering multiple sheets of it one on top of the other, involved folding graphene into three-dimensional spherical structures. Doing so creates countless tiny pores measuring 100 to 300 nanometers across. Electrons flow into the graphene, and air and lithium-ions can freely move about inside the graphene' s pores while the generated lithium peroxide is also stored in them. Using this method it has proved possible to achieve a capacitance 30 times higher than that of commercially available lithium-ion batteries. Unfortunately, since it is difficult for chemical reactions to take place in such material, an exces- sively high voltage is required during charging. As a result, the energy utilization efficiency (the ratio of the amount of electrical energy required to charge a battery against the amount of elec- trical energy that can be extracted from it when discharging), was only roughly 50%, and this poor performance prevented commercialization of the technology.
As a result of subsequent repeated experimentation, the team arrived at a graphene-based material with small amounts of added nitrogen and ruthenium etc. The material is called nitro- gen-doped nanoporous graphene, i.e. graphene with some carbon atoms replaced with nitrogen atoms and with ruthenium oxide (RuO2) nanoparticles trapped within. Using this material as a positive electrode provides a large maximum capacitance of 8,300mAh per electrode unit weight, and, since the material pro- vides a catalytic effect that accelerates chemical reactions, it also enables more rapid charging. The material has also demonstrat- ed an energy utilization ratio of over 72% and the fact that it can be charged and discharged 100 times or more means the materi- al represents a major step toward commercialization.
Schematic diagram of the surface of three-dimensional nanoporous graphene
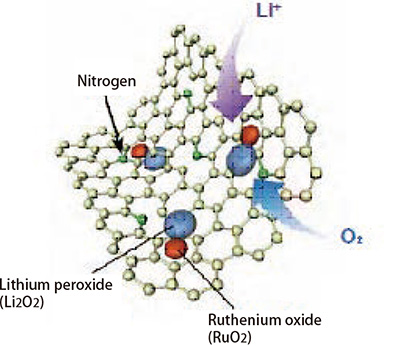
Some of the carbon atoms have been replaced with nitrogen atoms. The rutheni- um oxide trapped in the graphene acceler- ates chemical reactions.
Electron microscope photos of nanoporous graphene electrodes with trapped ruthenium oxide (RuO2) nanoparticles
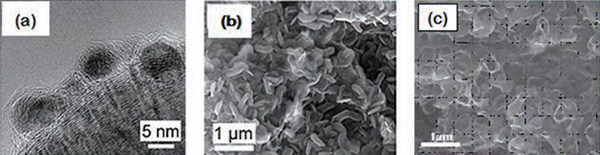
In photo (a) one can see the black agglomerations of ruthenium oxide trapped in the graphene sheets. Photo (b), in which the magnification has been reduced, shows the electrode prior to charging. The spaces in the graphene are large. After using this electrode to charge the battery, ruthenium oxide particles are stored in these spaces, as shown in photo (c), and the pores appear reduced in size. When charging and discharging the battery, the elec- trode repeatedly shifts between the states shown in photos (b) and (c).
Developing further technologies to move closer to commercialization
Professor Chen and his team will be working with companies and other organizations to make further progress with their research with the aim of eventually commercializing the technology. Increasing the size of the batteries to a commercially viable size is one of the issues they need to deal with. Although only a small amount of ruthenium is used, since it is an expensive material, the team is aiming to develop an alternative high-perfor-mance but low-cost catalytic material as well as other technologies to increase capacitance while also facilitating low overpo- tential.