Research Results
Elucidation of the principles behind RNA modification and pursuing research into its applications
Discovering the new functions of tRNA modification and unveiling its biological rolesFY2024

- SUZUKI Tsutomu (Professor, Graduate School of Engineering, The University of Tokyo)
- ERATO
- Research Director (2020-2025) of “ERATO SUZUKI RNA Modification Project”
The research group led by Professor Tsutomu Suzuki of The University of Tokyo is opening new horizons in RNA modification research. In particular, the group has discovered a reversible RNA phosphorylation, and tRNA glycosylation enzymes. These studies were published in top journals.
Reversible RNA phosphorylation contributes to cellular thermotolerance
Proteins and cellular metabolites are dynamically regulated by phosphorylation and dephosphorylation. This is a well-established fundamental concept in biochemistry and molecular biology. However, phosphorylation of RNA molecule had never been reported. The research group led by Professor Suzuki made the world-first discovery of reversible RNA phosphorylation in transfer RNAs (tRNAs) from thermophilic archaea and bacteria. The RNA phosphorylation contributes to thermal stability of tRNAs and confers resistance to nuclease, thereby contributing to cellular thermotolerance.
Discovery of tRNA phosphorylation
tRNA functions as an adaptor molecule to link the genetic code (codons) in mRNA with its corresponding amino acid during protein synthesis (translation). After transcription, tRNAs maturate through undergoing a variety of chemical modifications introduced enzymatically. These modifications contribute to accurate deciphering the cognate codons and the stability of tRNA core structure. Defects or abnormalities in tRNA modification result in dysregulation of gene expression, leading to pathological consequences. Protein phosphorylation is a well-characterized post-translational modification involved in various biological mechanisms including intracellular signaling pathways. However, internal RNA phosphorylation had never been discovered.
The research group isolated multiple species of tRNAs from the hyperthermophilic archaeon Sulfurisphaera tokodaii, analyzed their modification status by mass spectrometry combined with biochemical methods, and discovered a novel RNA modification at position 47 in the variable loop of tRNA. The chemical structure of the modification was determined to be 2’-phosphouridine (Up) in which the 2’ OH is phosphorylated (Figure 1A). The group measured melting temperatures (Tm) of the tRNA with and without the Up47 modification and revealed that the tRNA is thermally-stabilized with this single modification by 6.6°C of the Tm value. The group also found that Up47 has a role in protecting tRNA from nucleolytic degradation. In addition, structural study of tRNA using X-ray crystallography revealed that Up47 does not fix but flexibly maintains the tRNA core structure like a “padlock” by allowing a metastable structure during thermal denaturation of tRNA (Figure 1A). This is a completely novel mechanism to stabilize RNA structure by a post-transcriptional modification.
Cellular thermotolerance through tRNA phosphorylation and its reversibility
From thermophilic archaea and bacteria, the research group found a writer enzyme, named ArkI, which phosphorylates tRNA to introduce the Up47 modification (Figure 1B). Knockout of arkI gene in thermophilic archaea showed significant growth retardation at high temperature, indicating that Up47 modification of tRNA contributes to cellular thermotolerance of thermophilic organisms. Furthermore, the group identified an eraser enzyme called KptA which removes the phosphate group of Up47 from tRNA, and revealed that Up47 is a reversible modification in the cell (Figure 1B). This result indicates an epitranscriptomic regulation of gene expression in which tRNAs, like proteins, undergo functional regulation through the reversible phosphorylation. In extremophiles including thermophilic archaea and bacteria, the reversible Up modification might contribute to cellular survival at high growth temperature by dynamically modulating structural flexibility of tRNAs.
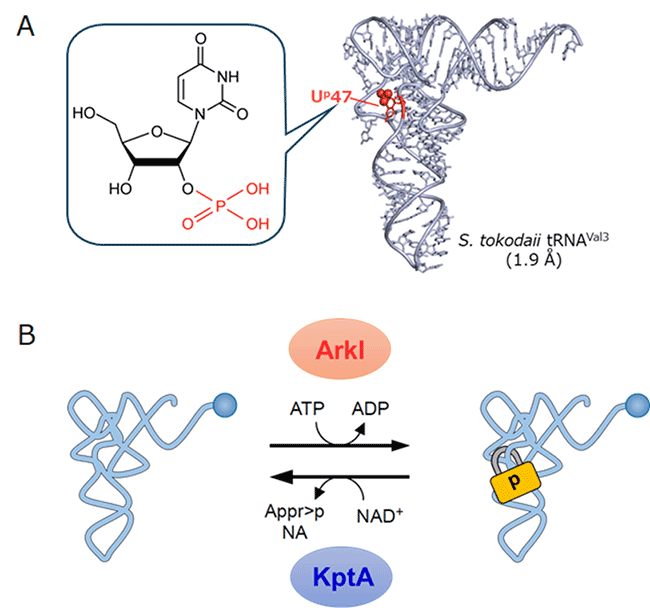
Fig.1. 2’-phosphouridine found in thermophilic archaeal
tRNA and its reversibility
(Ohira T, et al., Nature, 2022)
(A) The chemical structure of 2′-phosphouridine (Up) modification and crystal structure of S. tokodaii tRNAVal3 (resolution: 1.9 Å). The Up modification is shown in red. (B) Reversible tRNA phosphorylation by ArkI and KptA.
Glycosylation of tRNA regulates translation speed
tRNAs contain a variety of chemical modifications, which play an important role in protein synthesis. Recent studies have revealed that tRNA modifications are dynamically regulated in response to intracellular metabolites and environmental stresses, and that deficiency or misregulation of tRNA modification status is associated with various human diseases including mitochondrial diseases, neurological disorders, and cancer.
Queuosine (Q) (Figure 2), a guanosine derivative bearing a 7-deazapurine core structure with a cyclopentene diol group, is a unique tRNA modification found in many organisms from bacteria to humans. Since we, humans and vertebrates, cannot biosynthesize queuine (q), a base portion of Q, we have to take q as a micronutrient which is synthesized by gut microbiota or from food, so as to synthesize Q on tRNAs (Figure 2). In human and vertebrate, four species of cytoplasmic tRNAs contain Q and its derivatives. For tRNATyr, Q is further galactosylated to form galactosylqueuosine (galQ), whereas, for tRNAAsp, Q is mannosylated to form mannosylqueuosine (manQ) (Figure 2). These glycosylated Q modifications were discovered by Dr. Susumu Nishimura and his colleagues in 1976, but their biosynthesis and functions remained elusive for about half a century.
Discovery and function of Q-glycosylation enzymes
The research group identified two types of Q-glycosylation enzymes from rat and pig liver lysate by activity-based purification using ammonium sulfate fractionation and multistep column chromatographies. The enzyme that galactosylates tRNATyr to form galQ was named as QTGAL, while the other enzyme that mannosylates tRNAAsp to form manQ was named QTMAN (Figure 2).
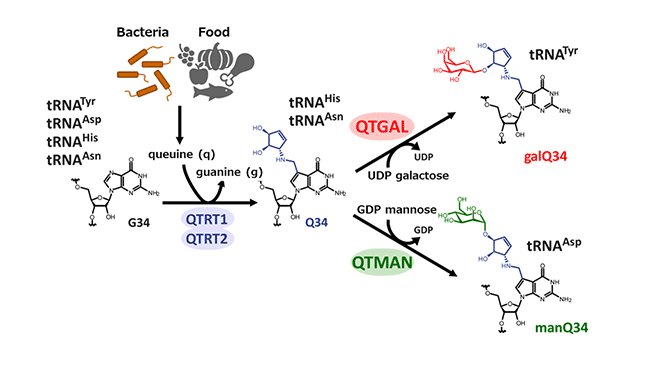
Fig.2 Biosynthesis of queuosine (Q) and glycosylated Q (Zhao X, et al., Cell, 2023)
Next, the group measured the translation speed by ribosome profiling. Compared to the wild type cell, the rate of UAC codons read by tRNATyr was faster in QTGAL knockout (KO) cells, while the rate of both GAC and GAU codons read by tRNAAsp was faster in QTMAN KO cells. These findings indicate that the Q-glycosylation plays a role in regulating the translation rate in a codon-specific manner.
Structural analysis of the ribosome-tRNA complex using cryo-EM (Figure 3A) revealed that the cyclopentene ring of Q recognizes the major groove of the codon-anticodon helix via hydrogen bond networks to facilitate codon recognition (Figure 3B). On the other hand, the glycosylations of Q weaken the effect of the major groove binder by rotating the cyclopentene ring (Figure 3CD). These structural findings nicely explain how Q-glycosylations regulate translation rate.
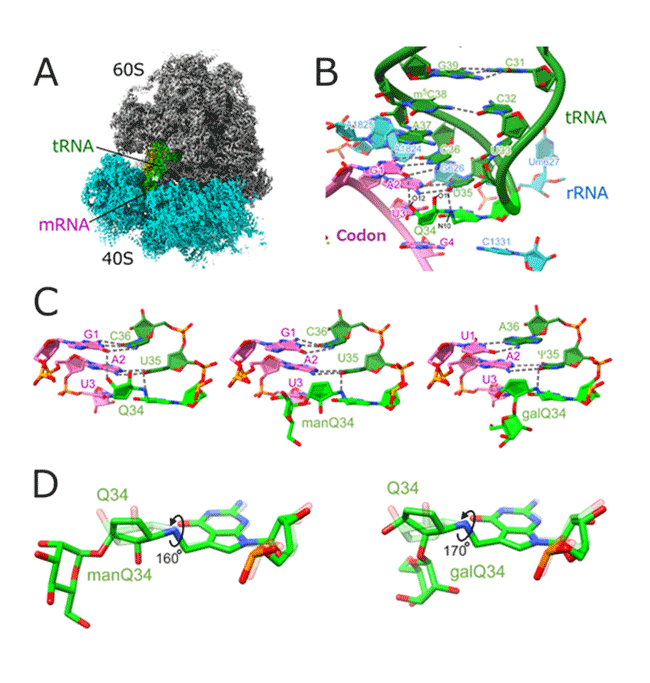
Fig.3 Glycosylated Q modification binds to the major groove
of codon-anticodon pairing
(Zhao X, et al., Cell, 2023)
(A) Cryo-EM structure of the human ribosome, mRNA, and tRNA complex. (B) Close-up view of codon-anticodon pairing. (C) Codon recognition mediated by Q, manQ, and galQ. (D) Rotation of cyclopentene ring by mannose and galactose addition.
Optimal translation mediated by tRNA modification plays a critical role in correct folding of nascent proteins and contributes to proteostasis. In fact, it was found that nascent proteins are prone to aggregate in QTGAL KO and QTMAN KO cells, indicating that Q-glycosylations play an important role in maintaining proteostasis (Figure 4).

Fig.4. Q-glycosylations regulate proper translation speed
and maintain proteostasis
(Zhao X, et al., Cell, 2023)
Physiological aspect of Q-glycosylation in zebrafish
Zebrafish were used to generate qtgal and qtman KO lines. These mutants normally developed but exhibited a significantly slower postnatal growth and short body length, compared to the wild type fish (Figure 4). Furthermore, phosphorylation of the translation initiation factor eIF2α was markedly enhanced in the qtgal KO lines, suggesting that perturbed translation rate and impaired protein folding might activate the integrated stress response. Taken together, these findings indicate that Q-glycosylations are required for efficient growth of zebrafish.
Future perspective for potential application of tRNA modifications in RNA therapeutics
RNA is an attractive modality in drug development, especially in the field of nucleic acid medicines including mRNA vaccines, siRNAs and aptamers. RNA drugs can be functionalized and stabilized by various types of tRNA modifications. tRNA modifications are also a key element to antagonize various RNA sensors to escape from innate immune system. The results obtained in this project are expected to be applied to RNA medicines in the future.
- Life Science
- Research Results
- Japanese