Research Results
Making large-scale optical quantum computers a reality
Method of flexibly controlling pulse waveforms of quantum light developedFY2023
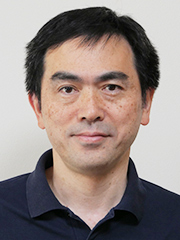
- FURUSAWA Akira (Professor of School of Engineering, the University of Tokyo, Deputy Director of RIKEN Center for Quantum Computing)
- Moonshot Goal 6: Realization of a fault-tolerant universal quantum computer that will revolutionize economy, industry, and security by 2050
- Project Manager (2020-2026), "Development of large-scale fault-tolerant universal optical quantum computers"
Succeeded for the first time in "generating quantum light with a special pulse waveform"
Quantum computers are believed to solve problems that would take even supercomputers an enormous amount of time to solve in the blink of an eye. Universal quantum computers are currently being studied in various types of systems, including atomic, ionic and superconducting devices, with the aim of realizing large-scale quantum computers. Among them, optical quantum computers,*1 which use light, are considered advantageous for practical use because they can operate at room temperature and in the atmosphere without the need for huge cooling or vacuum equipment required for other systems. Professor FURUSAWA Akira and Assistant Professor TAKASE Kan of the University of Tokyo in collaboration with research teams from Nippon Telegraph and Telephone Corporation (NTT), the National Institute of Information and Communications Technology (NICT) and RIKEN have proposed a light source that outputs any quantum light as a pulse waveform, the "Quantum Arbitrary Waveform Generator (Q-AWG)" (Fig. 1), and have implemented its core technology, a “method of flexibly controlling pulse waveforms of quantum light*2. They succeeded in "generating quantum light with a special pulse waveform," which is essential for the operation of a large-scale optical quantum computer.
The novel concept of Q-AWG in this study is a versatile quantum light source that outputs various pulsed quantum light depending on the purpose. Conventional light sources such as lasers and arbitrary waveform generators (AWGs) output only one type of light (classical light). In contrast, Q-AWGs can determine which quantum light to output from countless options, including various photon number states and the Schrodinger's cat state. In addition, the pulse waveform of the output quantum light can be set to the optimum one depending on the purpose. The "technology to flexibly control pulse waveforms of quantum light," which is at the core of Q-AWG, is demonstrated in this study.
*1 Optical quantum computer
While conventional computers perform calculations by representing information as either 0 or 1, quantum computers can hold multiple states of 0 and 1 and use this nature to instantly perform a number of different calculations. Many quantum computers use electrical signals. In contrast, optical quantum computers use optical signals, which have a high clock frequency and can perform calculations faster than electrical signals.
*2 Quantum light
A quantum is a very small amount of matter, faint light, or a unit of energy held by such a small entity. A quantum exhibits mysterious behavior that contradicts our intuition, such as taking on a "superposition" state in which multiple states coexist, and having the properties of both particles and waves. Light that strongly exhibits such characteristics unique to a quantum is called quantum light. In contrast to quantum light, light emitted from conventional light sources such as lasers is called classical light.
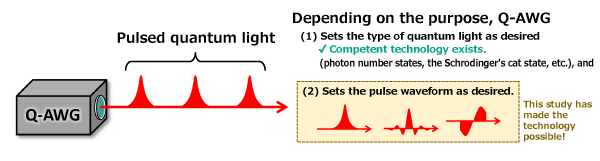
Fig. 1 Illustration of a Quantum Arbitrary Waveform Generator (Q-AWG)
A "quantum light source capable of outputting a variety of quantum light"
The invention of laser light has brought great advances in science and technology. One of the most versatile light sources in the modern era is an "arbitrary waveform generator (AWG)," which outputs laser light in an arbitrary pulse waveform. An AWG can be applied to a variety of applications, ranging from high-speed optical communications to control of materials at a molecular/atomic level, by optimizing the pulse waveform depending on the purpose. Although AWGs, which control light as desired, appear to be an excellent light source, they have a clear limitation of "being able to output only classical light, namely, laser light." This limits their use in the development of advanced quantum technologies.
Quantum technologies such as optical quantum computers, quantum networking and quantum measurement require a quantum light source that can output a variety of quantum light, including a "squeezed state, in which quantum noise of specific phase components is compressed,” a "photon number state, in which light is a wave but can be interpreted as a particle", and "Schrodinger's cat state,*3 in which classical light with different phases is superimposed." Designing pulse waveforms can provide numerous technological benefits also for quantum technology, as for classical light. Therefore, the realization of a "versatile quantum light source" that can output any kind of quantum light and flexibly control pulse waveforms, as in the case of AWGs, will greatly contribute to the progress of quantum technology.
*3 Schrodinger's cat state
The original meaning is the mysterious quantum-mechanical superposition of life and death of a single cat in Schrodinger's thought experiment. In optics, two classical lights of different phases are compared to a living cat and a dead cat, and their superposition is called the Schrodinger's cat state. The optical Schrodinger's cat state is known as an important resource for a variety of quantum technologies, including quantum computers.
"Principle of controlling pulse waveforms of quantum light using quantum entanglement" demonstrated
This study proposes a light source called a "quantum arbitrary waveform generator (Q-AWG)" that outputs arbitrary quantum light with an arbitrary pulse waveform, and develops a method to flexibly control pulse waveforms of quantum light, which is the core technology of the Q-AWG. This method was used to "succeed for the first time in generating quantum light with a special pulse waveform, which is essential for the operation of a large-scale optical quantum computer."
Aiming at the realization of a Q-AWG, the first step in this study was to devise a "method to control pulse waveforms while suppressing loss to quantum light." Classical light, such as laser light, does not change its physical properties when subject to loss, and can return to its original state using an optical amplifier. Therefore, an AWG that handles only classical light can easily control pulse waveforms by irradiating laser light to an optical filter with a large loss. In contrast, quantum light is highly susceptible to loss, and the unique physical properties of quantum light are rapidly lost. Moreover, quantum light that is subject to loss basically cannot return to its original state, making it unusable. Accordingly, the pulse waveform control of quantum light by a Q-AWG cannot use the method used for AWGs, which assumes large loss, requiring the introduction of a completely different methodology. To address this issue, in this study, a "new method of flexibly controlling pulse waveforms via quantum entanglement"*4 was devised.
The "principle of controlling pulse waveforms of quantum light using quantum entanglement" which was devised and demonstrated in this study is as follows.
When light 1 and light 2 are in quantum entanglement (Fig. 2), a targeted quantum state is generated on the light 1 side upon the detection of light after the quantum light 2 is irradiated to the photon detector.*5 An optical filter installed in front of the photon detector makes it possible to determine the pulse waveform of the quantum light to be generated.
In particular, "broadening the frequency bandwidth of light with quantum entanglement" increases the resolution of waveform control (the ability to measure the target), so that arbitrary pulse waveforms can be realized. This method eliminates the need to install an optical filter on the light 1 side where the target quantum light is actually generated, enabling the pulse waveform to be controlled while suppressing loss to the quantum light.
*4 Quantum entanglement
Mysterious correlations can arise between multiple quantum particles in the quantum world that cannot be explained by classical physics. Such correlations are called quantum entanglement. Quantum entanglement is also important in applications, and is a key resource in quantum communications and quantum information processing.
*5 Photon/photon detector
Quantum has a mysterious property of "having both particle and wave properties." Light was originally conceived as a wave, but quantum light can also be interpreted as a group of light particles. These light particles are called photons. A photon detector is a measuring instrument that determines whether the incident quantum light contains photons.
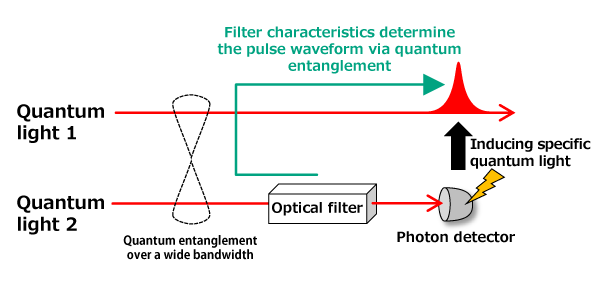
Fig. 2 Principle of controlling pulse waveforms of quantum light
The "broadband squeezed light source" jointly developed by the University of Tokyo and NTT, and the "superconducting photon detector" jointly developed by the University of Tokyo and NICT, were used to demonstrate the proposed method of controlling pulse waveforms (Fig. 3). In the experiment, squeezed light and a beam splitter (an optical device that splits the incident light by a predetermined split ratio) generated light with quantum entanglement, and by introducing an optical filter and a photon detector, quantum light in a state called the Schrodinger's cat state was successfully generated as a pulse with a balanced time-bin waveform (Fig. 4).
The large-scale optical quantum computer currently under development assumes the use of pulses with a balanced time-bin waveform (Fig. 4) so that multiple quantum lights do not adversely affect each other. No method for realizing a balanced time-bin waveform had been known, and this study is the first demonstration of such a method. This achievement demonstrates the high flexibility of the proposed method of controlling pulse waveforms, and its practicality in the development of quantum technology.
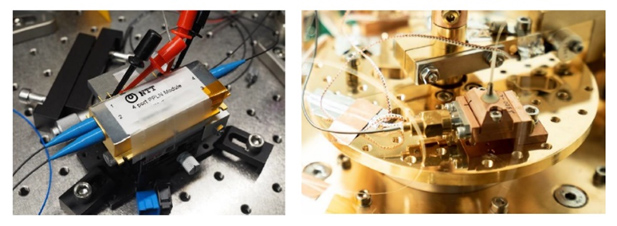
Fig. 3
Left: Broadband squeezed light source jointly developed by the University of Tokyo and NTT. It had previously been difficult to realize a broadband squeezed light source capable of outputting sufficient light intensity with low loss. Revamping the manufacturing process for the nonlinear optical crystal, which is the heart of the module, has greatly improved the performance, realizing a practical broadband squeezed light source, which was used also in this study.
Right: Superconducting nanostrip single-photon detector (SNSPD) jointly developed by the University of Tokyo and NICT. This study has been using the telecommunications wavelength band to generate high-purity broadband squeezed light, but the performance of conventional semiconductor photon detectors functioning in this wavelength band was unsatisfactory. A high-performance photon detector for the telecommunication wavelength band was designed to produce this device, which utilizes superconducting technology.
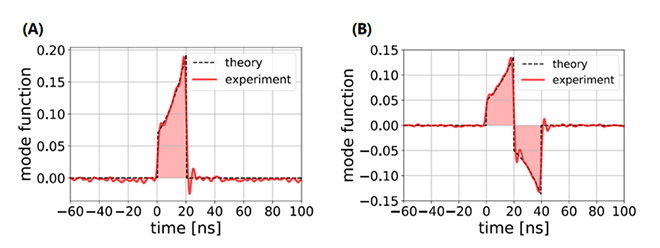
Fig. 4 Generated pulse waveform in the Schrodinger's cat state
A waveform in which a pulse is mostly within a finite region, as in (A), is called a time-bin waveform. A combined waveform of one time-bin waveform and its inverted form is called a balanced time-bin waveform (B). Quantum optical pulses having this waveform are highly efficient because they are continuous with no gap on a single optical axis, and neighboring pulses are less likely to adversely affect each other.
Moving the development of optical quantum computers to the next step
Extending the system developed in this study will help to generate general quantum light in addition to light in the Schrodinger's cat state, leading to the realization of a Q-AWG. The greatest value of Q-AWGs lies in their versatility. The realization of quantum technology requires a wide variety of quantum light and pulse waveforms, in the same way that an optical quantum computer requires a highly specialized pulse waveform. In addition, as research progresses, the requirements are constantly changing. A versatile quantum light source Q-AWG can flexibly respond to the needs of the times. The realization of a Q-AWG as an "ultimate quantum light source" through the application of this study is expected to promote the development of various quantum technologies, including optical quantum computers.