Research Results
Toward the realization of a universal quantum computer
Extending the research frontier of superconducting qubitsFY2023
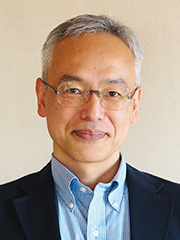
- NAKAMURA Yasunobu (Professor of Graduate School of Engineering, The University of Tokyo /Director of RIKEN Center for Quantum Computing)
- ERATO
- Research Director (2016-2021), "NAKAMURA Macroscopic Quantum Machines"
Established fundamental technologies for quantum control and measurement
Quantum computers largely outperform conventional computers in terms of efficient computation. They execute calculations by controlling and measuring the state of qubits, which are elementary devices carrying quantum information. How to physically implement qubits determines the computational scale of solvable problems and the accuracy of solutions and is crucial to realize a practically usable quantum computer.
The project led by Director NAKAMURA Yasunobu, who demonstrated the world's first qubit operation using a superconducting circuit in 1999, has been developing technologies related to superconducting qubits and quantum circuits based on them. The project focused on establishing techniques for high-fidelity control and measurement of quantum states using superconducting quantum circuit technologies.
Regarding wiring technology, which is one of the key challenges to realizing a large-scale quantum computer, the project team developed an architecture that enables “Integrated superconducting qubits in a dense two-dimensional array” to suppress crosstalk (leakage of signals to other wires) even when the system size increases. They succeeded, for the first time, in performing “quantum non-demolition measurement of single microwave photons”, which supports inter-module connection using microwave photons, useful for expanding and enhancing the functionality of qubit devices. They are also working on the construction of hybrid quantum systems*1, developed a new quantum sensor using a superconducting qubit, and succeeded in the detection of a single magnon using quantum entanglement*2.
*1 Hybrid quantum system
A system in which different physical degrees of freedom (various degrees of freedom in matters, electromagnetic fields, etc.) are coupled via interactions with each other, enabling quantum mechanical states to be transferred between them, and through this, allowing control and measurement that cannot be realized before.
*2 Quantum entanglement
A property of quantum-mechanically correlated states generated through interaction among two or multiple parties. Once a maximal quantum entanglement is established between two parties and the state of one party is determined by measurement, no matter how far apart they are, the state of the other party is instantly determined.
Various challenges confronting quantum computer research
1. Integrated superconducting qubits in a dense two-dimensional array
Establishing an architecture that can be applied to large-scale integrated circuits of qubits is a key challenge in circuit technology for superconducting quantum computers. Many research teams have adopted a method similar to that for semiconductor chips, which, however, leads to increasing wiring density with the number of qubits, resulting in problems such as an increase in crosstalk.
2. Quantum non-demolition measurement of a single microwave photon
New methods have been sought for the measurement and detection of electromagnetic waves and photons, as a fundamental technology for the control of quantum computers. So far, incident photons were always absorbed by detectors, making it impossible to use the detected photons at a later time. Therefore, “quantum non-demolition measurement”, in which only information on the presence or absence of incident photons is acquired and photons are reflected without being absorbed, was proposed. Although the measurement of near-infrared photons*3 was demonstrated in 2013, the measurement of microwave photons with four to five orders of magnitude smaller energy than near-infrared photons had not yet been realized before this project.
3. Detection of a single magnon
Constituting hybrid quantum systems, a platform that can control and detect photons as quanta of electromagnetic waves of various wavelengths and exchange their quantum states are anticipated in the quantum world. Magnons in ferromagnetic materials*4 are one of the candidates for the constituents. Magnons are the quanta of spin waves, which are the collective motion of electron spins in magnetic materials. Until now, due to the limited detection sensitivity, most methods have dealt with a large number of magnons, and there were no means to count magnons one by one.
*3 Near-infrared photon
Quantum of light with energy in the near-infrared region (with a wavelength of approximately 1 μm). Its energy is four to five orders of magnitude higher than that in the microwave region, allowing it to transfer quantum information even in a room-temperature environment.
*4 Ferromagnetic material
Electrons have the property of being microscopic magnets called “spins” analogous to their spinning motion. Magnetic materials exhibit magnetic ordering in which a macroscopic number of electron spins are ordered in some pattern and are classified into, e.g., ferromagnetic materials, in which the spins are aligned in a uniform direction and have magnetic poles as a magnet, and antiferromagnetic materials, in which adjacent spins are aligned in an antiparallel or mutually canceling manner and have no magnetic poles.
Taking quantum computer research one step further
1. Integrated superconducting qubits in a dense two-dimensional array
In this project, they designed and developed a new architecture in which the wiring density remains the same and crosstalk is suppressed even when the number of qubits increases. They used a method in which the qubits are accessed vertically by electrodes and coaxial cables penetrating the substrate. They also established a process technology for 16- and 64-qubit circuits (Fig. 1) based on the architecture and conducted prototyping and evaluation experiments. Single- and two-qubit gates and frequency-multiplexed qubit readouts were implemented on the integrated qubits in a dense two-dimensional array.
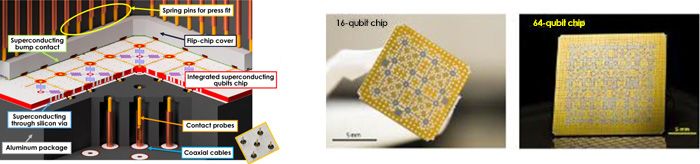
Fig. 1 Schematic image of integrated superconducting qubits and their packaging (left), and prototypes of a qubit chip (right)
2. Quantum non-demolition measurement of single microwave photons
The research group coupled a transmission line (coaxial cable) through which incoming microwave photons propagate and a superconducting qubit via a microwave cavity resonator (Fig. 2). First, an appropriate superposition of the ground and excited states (called the “+” state) is prepared as the initial state of the superconducting qubit. When a single microwave photon arrives and is reflected by the resonator, the superposition state of the qubit placed inside the resonator changes, and the qubit takes a state orthogonal to the initial state (called the “-” state) (Fig. 3). It is possible to know whether or not a microwave photon has arrived by observing whether the superconducting qubit is in the “+” state or the “-” state right after that. Since the incident photon is reflected and continues to propagate, quantum non-demolition measurement is realized. Using a highly sensitive Josephson parametric amplifier*5, the research team realized a microwave photon detection efficiency of 84% and further confirmed experimentally that the detected photons are reflected without being destroyed, thereby demonstrating quantum non-demolition measurement for the first time.
*5 Josephson parametric amplifier
An amplifier using the phenomenon of "parametric amplification", which occurs, for example, when circuit parameters such as coils and capacitors are modulated at twice the resonant frequency of a resonant circuit, is called a parametric amplifier. A parametric amplifier using a Josephson junction (a circuit element in which two superconductors are weakly coupled via an insulating tunnel barrier) leads to parametric amplification by modulating the effective inductance of two parallel Josephson junctions with a microwave magnetic field. This method provides signal amplification with very high gain and low noise.
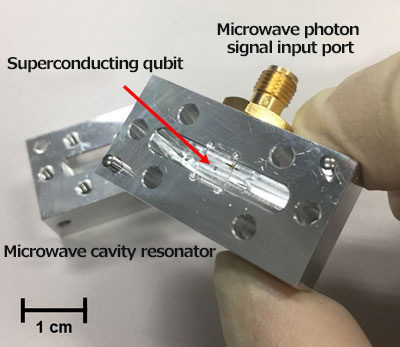
Fig. 2 Superconducting qubit coupled with a microwave cavity resonator
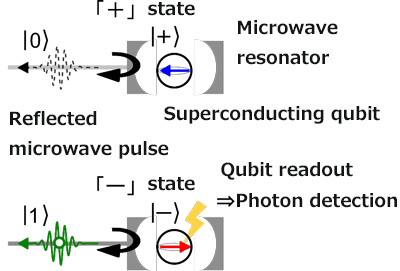
Fig. 3 Principle of a quantum non-demolition single microwave-photon detector using a superconducting qubit
When a single microwave photon is reflected by the resonator, the superposition state of the ground and excited states of the superconducting qubit inside the resonator changes from the initial state (“+” state) into a state orthogonal to the initial state (“-” state).
3. Realization of single-shot readout of a single magnon
Until now, due to the limited detection sensitivity, experimental studies on magnons have dealt with states in which a large number of magnons are excited, and there were no means to count them directly. In this project, they realized for the first time a technique for single-shot measurement (rather than an average of multiple measurements) of the state in which a single magnon is excited in a ferromagnetic material using a superconducting qubit placed in the same microwave resonator (Fig. 4).
Specifically, by using the interaction between magnons in a ferromagnetic single crystal of yttrium iron garnet (YIG) and a superconducting qubit mediated by a microwave resonator, they realized a condition called the strong dispersion limit, where the resonance energy of the superconducting qubit shifts depending on the number of magnons, and succeeded in the single-shot measurement of a single magnon with a high detection efficiency of 71%.
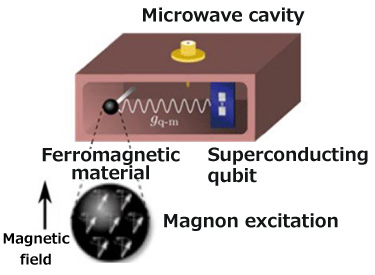
Fig. 4 Single-magnon detector
For single-magnon detection, A magnon in a magnetic single-crystalline sphere is coherently coupled with a superconducting qubit via a microwave cavity resonator.
Potential for establishing foundations and developing applications in each area
The activities of the research group have brought remarkable academic and industrial impacts to the acceleration of research and development of superconducting quantum computers.
1. Integrated superconducting qubits in a dense two-dimensional array
Towards the realization of large-scale integrated quantum computing circuits, a prototype of a 64-qubit circuit has been developed, and the evaluation has started. These are also the technical basis of the "Q-LEAP Flagship Project", a research and development program aiming for discontinuous solutions (quantum leap) to address important economic and social issues by making full use of quantum science and technology (optical and quantum technologies).
2. Quantum non-demolition measurement of a single microwave photon
The results of this study are essential for quantum network technology, in which quantum information is exchanged between qubits on superconducting circuits. The development of circuit components to control the propagation of microwave single-photons and manipulate their states will lead to applications to quantum networks and large-scale distributed quantum computers are expected. Single photon detectors with high sensitivity and small dark count*6, which did not exist in the microwave region, are expected to be applied to various quantum sensing techniques.
3. Realization of single-shot readout of a single magnon
The successful development of a single magnon detector is one of the beautiful examples of how superconducting qubits are not only a basic element of quantum computers, but also a breakthrough in the field of quantum sensing. It is expected to accelerate experimental research on quantum control and measurement of collective spin excitations in magnetic materials at the level of a single magnon and to be a cornerstone in paving the way for the generation of non-classical magnon states, which has not been realized yet.
*6 Dark count
The number of false signals erroneously generated when no photons are arriving.