Research Results
Aiming for the realization of next-generation computers
World's first successful fabrication of semiconductor spin quantum dots (artificial atoms)FY2023
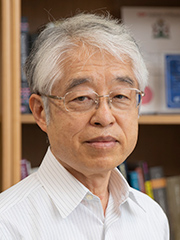
- TARUCHA Seigo (Group Director, Center for Emergent Matter Science, RIKEN)
- CREST
- Research Director(2016-2022) "Development of base technologies for spin-based quantum computing" in the research area of "Creation of an innovative quantum technology platform based on the advanced control of quantum states"
World's first demonstration of high-precision universal operation
The research team demonstrated high-precision universal operations*1 using electron spins*2 in a silicon quantum dot device, which is the smallest unit of information in a silicon quantum computer*3 (Fig. 1). For the first time in the world, they succeeded in freely operating two electron spins with a high degree of precision that allows "quantum error correction", which eliminates errors made by quantum computers.
This achievement provides a guideline for the realization of "quantum error correction", which is one of the challenges for the practical application of quantum computers using silicon quantum dots, and is expected to accelerate research and development in the future. This study was published in Volume 601 of Nature, a world-class scientific journal, and attracted a great deal of attention, with an image symbolizing these efforts on its cover.
*1 Universal operations
The basic set of operations that make up a quantum operation, consisting of a single-qubit operation and a two-qubit operation. All quantum operations can be realized by combining elements of the universal operations.
*2 Electron spin
Internal degrees of freedom of rotation in which an electron rotates clockwise or counterclockwise.
*3 Quantum computer
A computer that uses "superposition" in quantum mechanics to achieve massively parallel computing. Quantum algorithms have been developed that can solve problems such as factorization in a few hours, which used to take astronomical amounts of time with conventional computers, and are expected to enable ultra-high-speed computing.
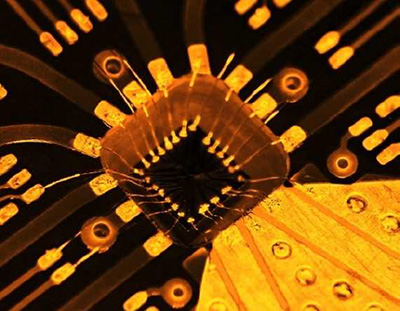
Fig. 1 The silicon quantum computer chip used in the study
"Quantum error correction" for qubits, a barrier to practical use
Computers have continued to evolve by improving information processing capability through miniaturization of semiconductor devices. In recent years, however, the physical limits of miniaturization have approached, and there are calls for the practical application of next-generation computers that perform calculations with non-conventional operating principles. Among these, the most promising is the quantum computer, which utilizes the phenomena of quantum mechanics to perform parallel computations at ultra-high speeds that exceed those of supercomputers.
Various types of quantum computers are currently being studied, but silicon quantum computers using silicon quantum dot*4 devices are considered to be suitable for large-scale quantum computer implementation because of their compatibility with existing semiconductor industry integration technologies. To enable quantum computers to perform calculations on a large scale, however, quantum bits (the smallest unit of information handled by a quantum computer) must overcome the challenge of losing information due to noise generated by impurities, heat, and other factors. For these reasons, R&D is underway in many countries to implement "quantum error correction", by a circuit that corrects errors that occur during the operation of a quantum computer. Implementing the most basic "quantum error correction" requires at least three qubits. In silicon quantum computers, basic operation of up to two qubits has been possible so far, but it has been difficult to fully control more than three qubits with high precision.
*4 Quantum dot
A zero-dimensional structure in which the motion of electrons is restricted by confining them in all three spatial directions. It is also called an artificial atom because of this property, and electrons can move in and out one by one.
Three-qubit gate confirms quantum error correction
To demonstrate that fault-tolerant silicon quantum computers can be realized, the research team first investigated silicon materials for qubits. Then, a high-purity crystal of a silicon isotope (28Si) without nuclear spin was adopted for the substrate. The substrate consisting of Si sandwiched by silicon germanium (SiGe) was micromachined, and electron spins were confined by applying a positive voltage from an aluminum fine gate electrode. Further, quantum dots capable of controlling and operating the electron spin were fabricated to reduce magnetic field noise that affects data in quantum computers, and the quantum coherence time*5 was extended to the microsecond level to resolve the condition that caused errors. In terms of error correction, which corrects errors that occur while a quantum computer is operating, the high precision of universal operations on one or two qubits, which serve as basic logic gates, was improved to more than 99% in all processes, including data readout and initialization, thereby clearing the fault-tolerance threshold (Fig. 2).
In addition to demonstrating high-precision error correction in silicon quantum dots, this study also demonstrated high-precision quantum calculations using two qubits, showing that fault-tolerant silicon quantum computers are feasible.
In a qubit device using electron spins in a silicon quantum dot device, the realization of "quantum error correction" at three qubits was also investigated. Using a microfabricated quantum dot structure (Fig. 3) on a silicon/silicon germanium semiconductor base, which is common in silicon quantum computers, the research team succeeded in forming quantum dots with a high degree of freedom by controlling the voltage applied to the gate electrode, and controlling the state of their electron spins.
Besides quantum gates with up to two qubits, technologies necessary for quantum error correction were confirmed for three-qubit gates, including "correcting the state of a data qubit based on the detected error", "detecting a phase error that occurred in any one of the three qubits", and "correcting a data qubit to its state before the error occurred".
*5 Coherence time
The typical time that a qubit maintains information. The information in a qubit, which is encoded using a quantum-mechanical superposition state, is affected by noise from the outside world and other sources, and is usually lost over time. Therefore, to operate a qubit accurately, the coherence time must be long enough to exceed the operation time.
*6 Fidelity
A figure of merit that shows how close a qubit's operation is to its ideal operation. A value of 100% indicates perfectly ideal operation, and a value of 99% or more is needed to realize a quantum computer with realistic quantum error correction.
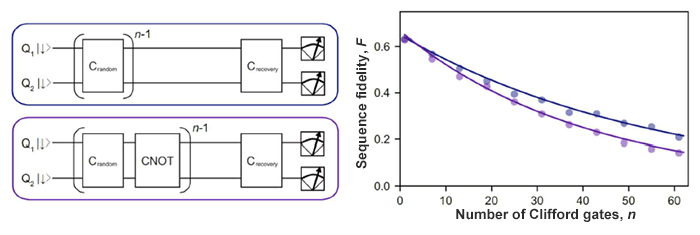
Fig. 2 Evaluation of operational fidelity*6 in two qubits (random benchmark method)
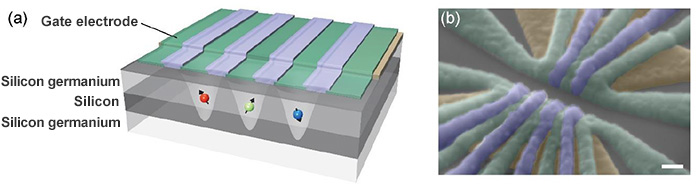
Fig. 3 Silicon/silicon germanium quantum dot sample
(a) Schematic diagram of three qubits. By controlling the voltage of the gate electrode, the electrons are confined in the silicon layer precisely, one by one.
(b) Electron micrograph of three qubits. The scale bar is 100 nanometers (1 nanometer is 1/1 billion meters).
Further research based on semiconductor microfabrication technology
Silicon quantum computers have good compatibility with existing semiconductor integrated circuit technology, and are expected to lead to the realization of a large-scale quantum computer with 100 million bits integrated on a single chip in the future. The research team plans to confirm the operation of "quantum error correction" on a scale of several tens of qubits, and to extend the research in cooperation with semiconductor manufacturers and other players.
Since Japan stands at the world's leading edge in semiconductor microfabrication technology, it is expected to leverage this foundation to become a pioneer in the technology that supports error-correcting silicon quantum computers, and to become a frontrunner in the field of quantum computers.