Hybrid Quantum Systems
Research overview
Radio waves from a radio station, visible light from the sun, and x-rays used in hospitals, are all electromagnetic waves. The electromagnetic waves propagate at the speed of light by oscillating electric and magnetic fields alternately. The electromagnetic waves are the most important elements in the information era, where they are deployed in the right place. For instance, microwaves with the wavelength of a few centimeters carry voice information on phone calls. Infrared light waves with the wavelength of a few micrometers, on the other hand, transfer information on the internet via optical fiber. As the energy of the electromagnetic wave decreases toward communicating with smaller energy, the particle aspect of the electromagnetic wave shows up. Eventually, the electromagnetic wave becomes photons, for which we can count as one, two... Mysterious is the photons’ nature: The photons exhibit particle nature as well as their original wave nature! Our intention is to study “quantum hybrid systems” which act as platforms for measuring, controlling, and exchanging “photons with vastly different wavelengths” in a “quantum world” where the particle and wave natures of photons intervene.
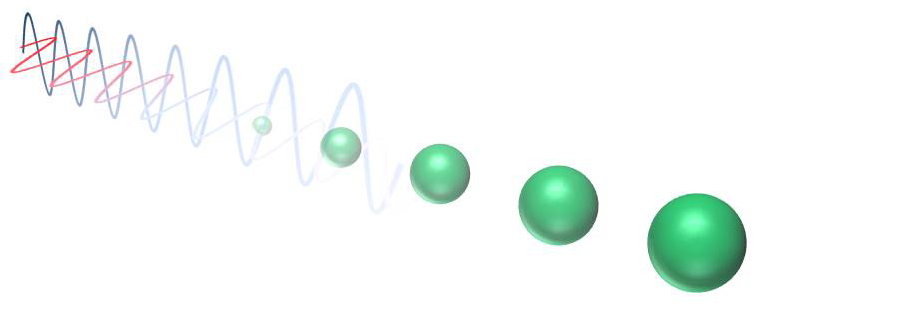
Three questions
The following are three questions to help understand our intention:
Q1: Why “Quantum world”?
- To deal with weak electromagnetic waves aiming at realizing energy-efficient communication, the “quantum world” reveals itself.
- It is possible to realize physically secure communication in the “quantum world” since quantum states are destroyed when someone observes them.
- Quantum computers, which compute in the “quantum world”, can be networked when they are connected through quantum nodes which also work in the “quantum world”.
Q2: Why “Photons with vastly different wavelengths” are needed?
- The characteristics of the electromagnetic waves differ and thus the available equipment for them are largely different. For instance, there are good lasers, high sensitive detectors, and low-loss fibers for infrared light. These facts make the infrared light suitable for long-distance communication. On the other hand, for microwaves, phase-stable sources, good mixers, and low-noise amplifiers are available, and thus the amplitude and phase are independently and accurately controlled. Superconducting quantum devices are thus controlled by these microwave equipment.
- There are a plethora of phenomena where electromagnetic waves with vastly different wavelengths are involved. For example, fluorescence from Na atoms is colored orange and involves photons with visible wavelengths, while ground-state hydrogen atoms radiate 21-cm wavelength radio waves. As for the magnetic resonances, proton nuclear magnetic resonances that spin in water can be resonantly excited by 40-MHz radio waves under a 1T magnetic field, while electrons spin in a ferromagnetic material, Yttrium Iron Garnet, can be excited by a 10-GHz microwave under a 0.3T magnetic field.
Q3: What are “Quantum hybrid systems”?
- We need matter to control electromagnetic waves since there is almost no interaction purely between electromagnetic waves. The stronger the interaction between electromagnetic waves and matter is even more pressing when the weaker electromagnetic waves are dealt with in the “quantum world”. To this end we use a cavity to localize and enhance the electromagnetic waves.
- When the interaction between an electromagnetic wave and a collective excitation in a matter is strengthened to a large extent by a cavity, the energy is exchanged between them. The total system becomes a “hybrid quantum system” where the entity is neither purely electromagnetic nor collective-excitation but is a mixture.
- “Hybrid quantum systems” will act as quantum nodes of the futuristic quantum internet, the internet operating in the “quantum world”.
Examples of hybrid quantum systems
We are interested in a variety of hybrid quantum systems. The following three are the examples:
Mechanical oscillators
Electromagnetic waves interact with matter via radiation pressure. The radiation pressure can be seen in an ultra-wide spectrum of electromagnetic waves, thus it is useful in transducing signals between electromagnetic waves with vastly different wavelengths. We are, for example, trying to optically readout a feeble radio-frequency signal of nuclear magnetic resonance via a mechanical oscillator.
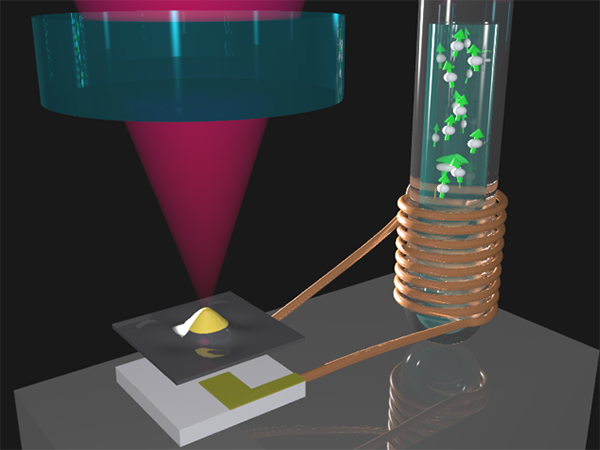
Surface acoustic waves
Electromagnetic waves interact with mechanical degrees of freedom not only via radiation pressure, but also through piezoelectric effects and photo-elastic effects. Exploiting the latter effects, we are especially interested in surface acoustic waves that are localized in the vicinity of the material surface. The aim is to transduce quantum information within microwaves, surface acoustic waves, and light waves.
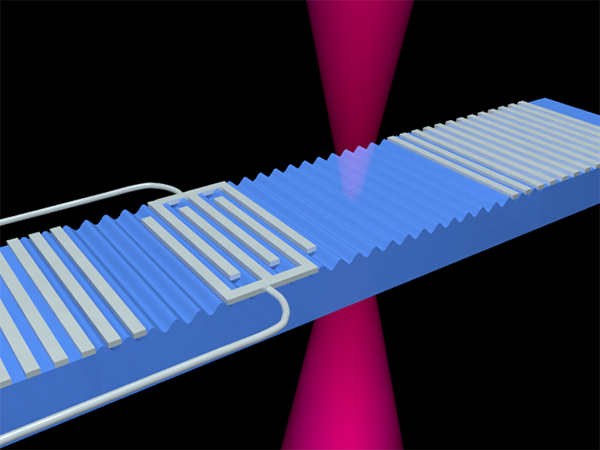
Ferromagnets
Magnetic matter, like ferromagnets, interacts with the magnetic component of electromagnetic waves via a magnetic dipole interaction. They also interact with the electric component of electromagnetic waves through an electric dipole interaction with a spin-orbit interaction. By utilizing a cavity, we are exploring the boosted interaction between magnets and electromagnetic waves to open up a new field called cavity magnonics.
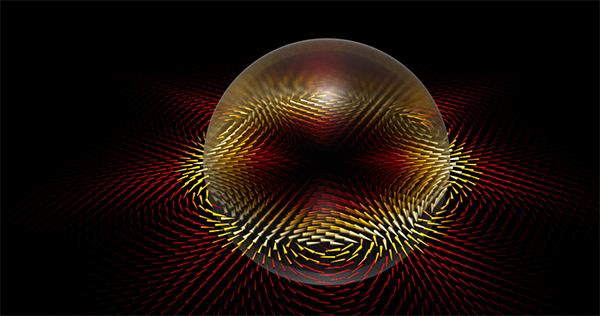